Can you tell us about your career before coming to the University of Maryland in 2020?
I began my career in 2014 on active duty in the United States Air Force as a full-time radio communications technician at the Royal Air Force Croughton in the United Kingdom. I coordinated communications to the battlefield by maintaining a fixed satellite communication relay station. This involved building a range of skills in electronics repair, network testing and operations management. In 2019, I chose to cross-train to a reserve aircraft maintenance position at the 459th Air Refueling Wing in Joint Base Andrews, Maryland. I inspected, serviced, fueled and launched our fleet of KC-135 refueler aircraft to support long-range missions.
Serving gave me the opportunity to grow as a professional in several exciting fields. I am immensely thankful for my many family members, mentors, and fellow servicemen and women who encouraged me to pursue my bachelor’s full-time at UMD starting in fall 2020.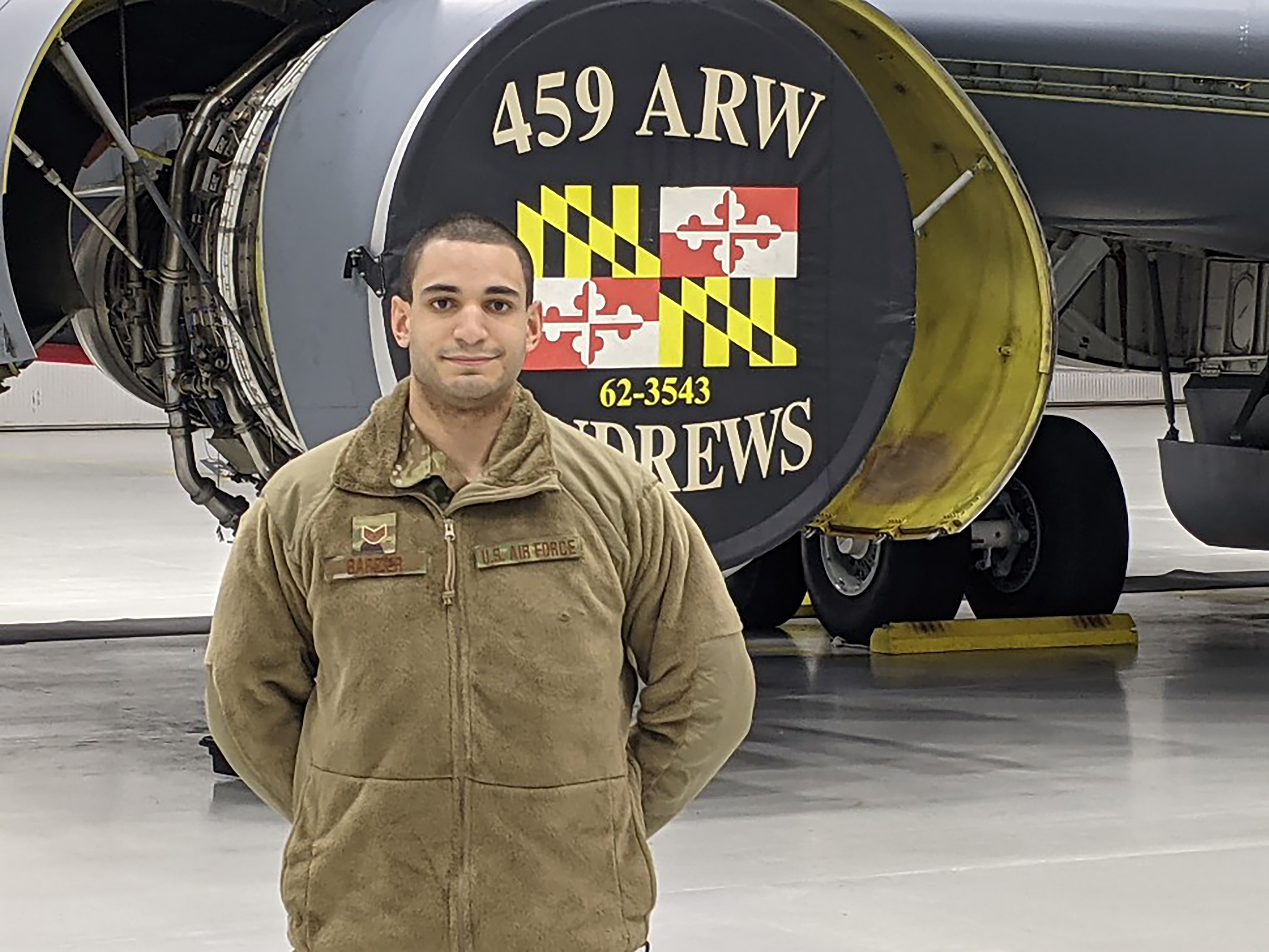
Why did you decide to study physics at UMD and what do you hope to do with your degree?
Since I was a kid playing with circuit kits and disassembling items around the house, I’ve been drawn to technical fields. When I was working in the military, I found myself pondering deep questions about the nature of reality. As a technician, I spent my time learning how to use the equipment; I wanted to know how and why everything worked. I was curious about everything from quantum particles to the behavior of black holes.
In my travels as a service member, I experienced the world in ways I’d only heard or read about. I saw issues like resource scarcity and environmental degradation everywhere I traveled and decided that I wanted to be part of the solution.
This led me to build an interest in the potential of nuclear fusion power (for energy and space travel). I decided to go into research to move this field forward while learning the intricacies of particles and the current state of reactors. My ultimate goal is to solve energy and environmental challenges with sustainable power sources.
How have you taken advantage of opportunities on campus to pursue your career goals?
First off, from the Terp Vets community, I have found support from other ambitious Terps who transitioned from the military to higher education.
Then in the University Career Center, I made use of essential guidance and career prep resources. After some searching into research projects to join, I found a promising one at the intersection of both CMNS and the Clark School of Engineering. I joined a research team in beam physics at the Institute for Research in Electronics and Applied Physics. Here, I developed my ability to use tools for constructing and testing elements of an electron accelerator. Across the board, I have had so much support from UMD interested in seeing me succeed.
What kind of career guidance and one-on-one feedback did you receive from the University Career Center @ CMNS?
At the Career Center, I spoke with [University Career Center @ CMNS Program Director] Becca Ryan who helped me understand my goals and prepare for internships. In our first meeting, Becca informed me that physics is a degree with many marketable skills, like analysis and research, that can match well to a range of internships and job postings.
After attending the university’s spring career fair and interviewing with a company of interest, UCC counselors encouraged me by suggesting the skills I would need to succeed and coaching me through the unfamiliar parts of the process such as negotiation.
As I interviewed, there were questions specific to the industry that I was unsure how to answer. Becca recommended studying material beforehand and asking staff members about the company’s priorities. She also helped me decide what to do with the job offer I received and determine how the job aligned with my goals and career path. Once I made my decision to decline the job offer and pursue graduate studies instead, Becca helped me vocalize it clearly and effectively.
What do you think your next stop after graduation will be (or what do you hope it will be)?
Now that I have graduated with my B.S. in physics, I hope to build upon my physics background and connect to engineering and business to solve needs in the world through products or a startup. I am currently enrolled in the accelerated business master’s program (here at UMD!) to combine my scientific and engineering skills with industry and leadership. It’s a one-year program that will give me the business, financial and communication skills so I can develop technologies that can reach the marketplace.
Next summer, I’ll be looking to gain internship experience this summer and apply for jobs before graduating with my master’s.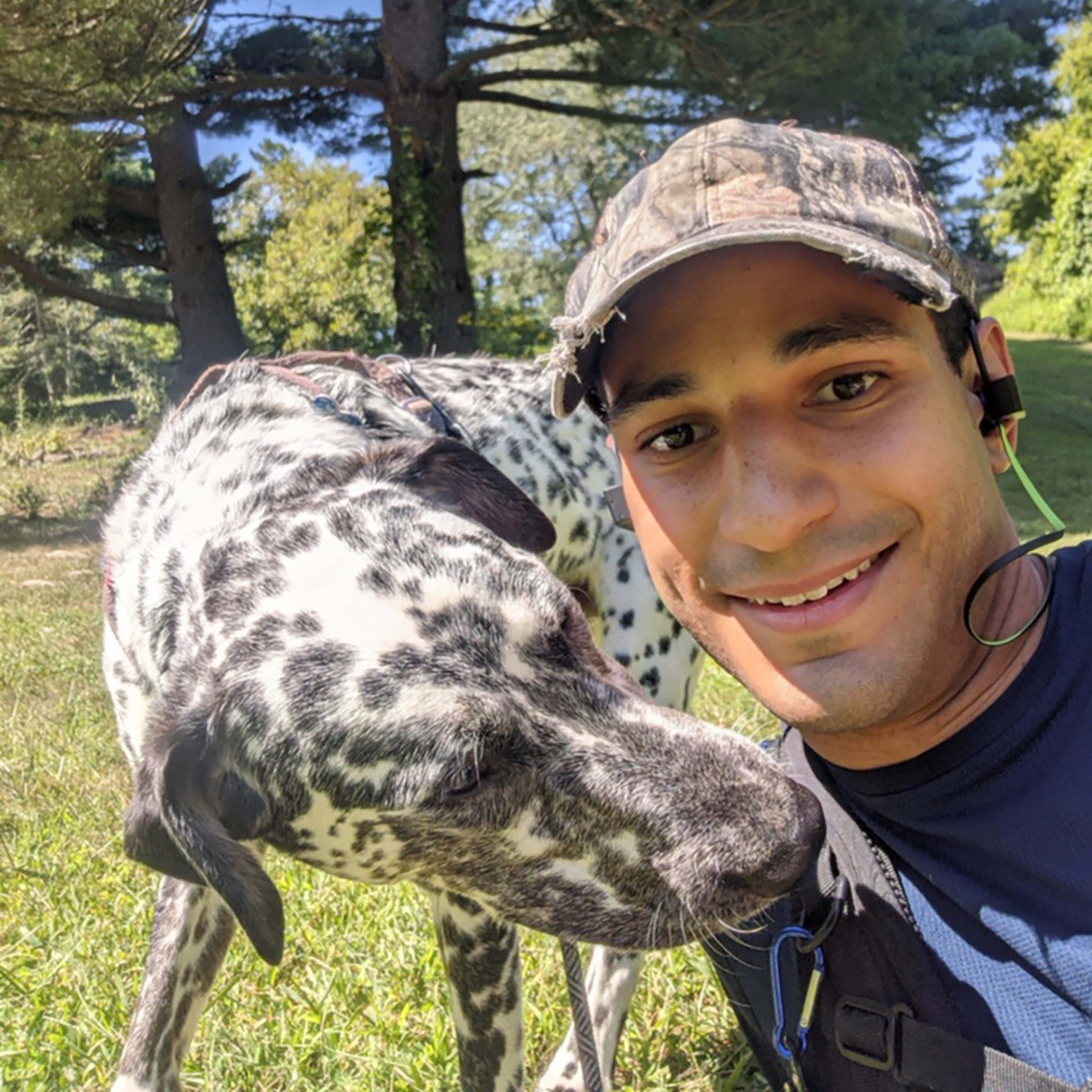
What advice do you have for fellow Science Terps who are looking for internships and jobs?
I am honored by the opportunity to be a Terp and study in such an encouraging and idea-abundant environment! I encourage other Science Terps to speak with the UCC and meet with employers and labs. You never know what skills of yours are in demand until you get yourself out there.
CMNS students have access to career advisors and programs that are personalized to their unique career interests in STEM fields. In this Q&A series, we are spotlighting how Science Terps are capitalizing on the resources, support and guidance that the University Career Center @ CMNS provides.
Make an appointment with Becca or another member of the University Career Center team by visiting umd.joinhandshake.com or email This email address is being protected from spambots. You need JavaScript enabled to view it. with any career-related questions!