New Study Identifies Mechanism Driving the Sun’s Fast Wind
- Details
- Category: Research News
- Published: Wednesday, June 07 2023 10:46
The fastest winds ever recorded on Earth reached more than 200 miles per hour, but even those gusts pale in comparison to the sun’s wind.
In a paper published June 7, 2023 in the journal Nature, a team of researchers used data from NASA’s Parker Solar Probe to explain how the solar wind is capable of surpassing speeds of 1 million miles per hour. They discovered that the energy released from the magnetic field near the sun’s surface is powerful enough to drive the fast solar wind, which is made up of ionized particles—called plasma—that flow outward from the sun.
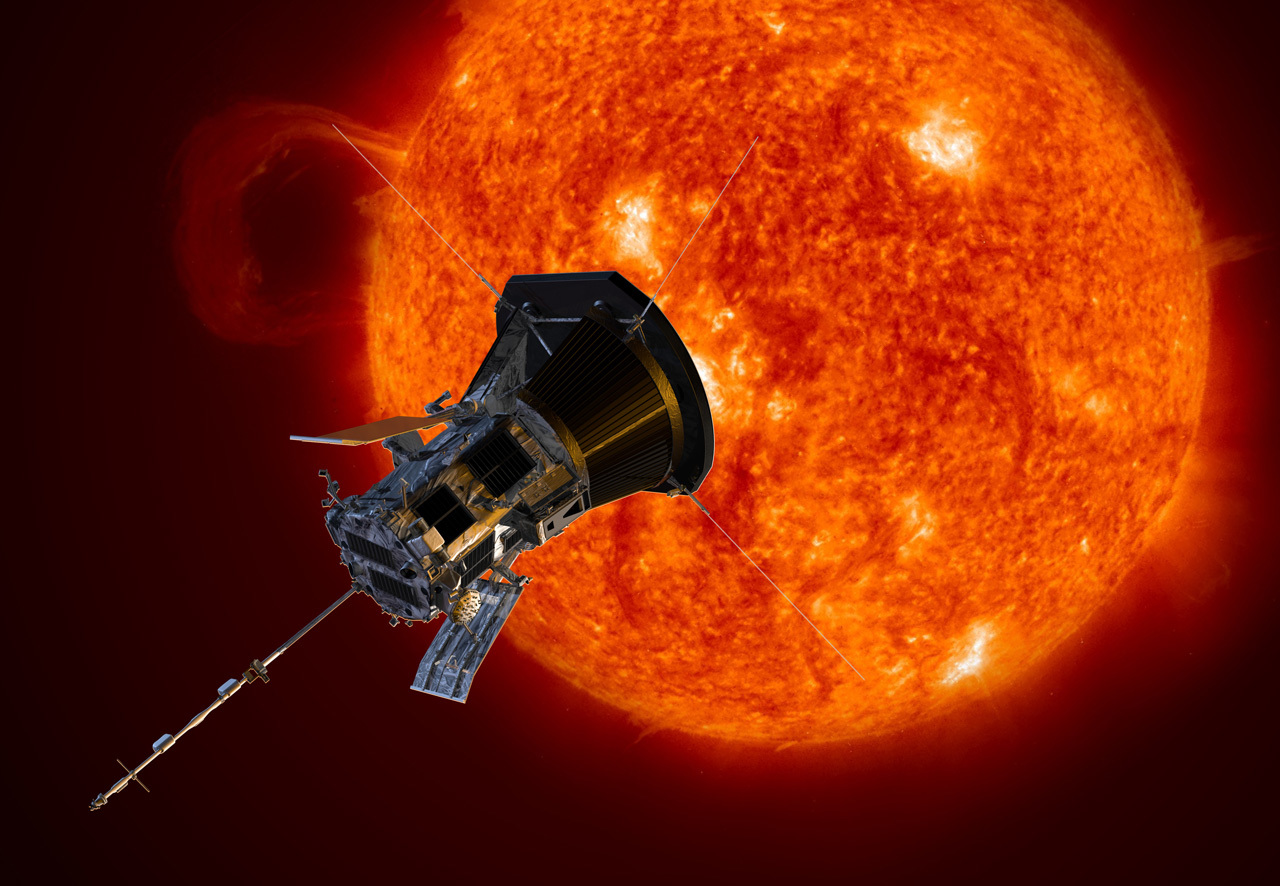
James Drake, a Distinguished University Professor in the University of Maryland’s Department of Physics and Institute for Physical Science and Technology (IPST), co-led this research alongside first author Stuart Bale of UC Berkeley. Drake said scientists have been trying to understand solar wind drivers since the 1950s—and with the world more interconnected than ever, the implications for Earth are significant.
The solar wind forms a giant magnetic bubble, known as the heliosphere, that protects planets in our solar system from a barrage of high-energy cosmic rays that whip around the galaxy. However, the solar wind also carries plasma and part of the sun’s magnetic field, which can crash into Earth’s magnetosphere and cause disturbances, including geomagnetic storms.
These storms occur when the sun experiences more turbulent activity, including solar flares and enormous expulsions of plasma into space, known as coronal mass ejections. Geomagnetic storms are responsible for spectacular aurora light shows that can be seen near the Earth’s poles, but at their most powerful, they can knock out a city’s power grid and potentially even disrupt global communications. Such events, while rare, can also be deadly to astronauts in space.
“Winds carry lots of information from the sun to Earth, so understanding the mechanism behind the sun’s wind is important for practical reasons on Earth,” Drake said. “That’s going to affect our ability to understand how the sun releases energy and drives geomagnetic storms, which are a threat to our communication networks.”
Previous studies revealed that the sun’s magnetic field was somehow driving the solar wind, but researchers didn’t know the underlying mechanism. Earlier this year, Drake co-authored a paper which argued that the heating and acceleration of the solar wind is driven by magnetic reconnection—a process that Drake has dedicated his scientific career to studying.
The authors explained that the entire surface of the sun is covered in small “jetlets” of hot plasma that are propelled upward by magnetic reconnection, which occurs when magnetic fields pointing in opposite directions cross-connect. In turn, this triggers the release of massive amounts of energy.
“Two things pointing in opposite directions often wind up annihilating each other, and in this case doing so releases magnetic energy,” Drake said. “These explosions that happen on the sun are all driven by that mechanism. It’s the annihilation of a magnetic field.”
To better understand these processes, the authors of the new Nature paper used data from the Parker Solar Probe to analyze the plasma flowing out of the corona—the outermost and hottest layer of the sun. In April 2021, Parker became the first spacecraft to enter the sun’s corona and has been nudging closer to the sun ever since. The data cited in this paper was taken at a distance of 13 solar radii, or roughly 5.6 million miles from the sun.
“When you get very close to the sun, you start seeing stuff that you just can’t see from Earth,” Drake said. “All the satellites that surround Earth are 210 solar radii from the sun, and now we’re down to 13. We’re about as close as we’re going to get.”
Using this new data, the Nature paper authors provided the first characterization of the bursts of magnetic energy that occur in coronal holes, which are openings in the sun’s magnetic field as well as the source of the solar wind.
The researchers demonstrated that magnetic reconnection between open and closed magnetic fields—known as interchange connection—is a continuous process, rather than a series of isolated events as previously thought. This led them to conclude that the rate of magnetic energy release, which drives the outward jet of heated plasma, was powerful enough to overcome gravity and produce the sun’s fast wind.
By understanding these smaller releases of energy that are constantly occurring on the sun, researchers hope to understand—and possibly even predict—the larger and more dangerous eruptions that launch plasma out into space. In addition to the implications for Earth, findings from this study can be applied to other areas of astronomy as well.
“Winds are produced by objects throughout the universe, so understanding what drives the wind from the sun has broad implications,” Drake said. “Winds from stars, for example, play a crucial role in shielding planetary systems from galactic cosmic rays, which can impact habitability.”
This would not only aid our understanding of the universe, but possibly also the search for life on other planets.
###
In addition to Drake, Marc Swisdak, a research scientist in UMD’s Institute for Research in Electronics and Applied Physics, co-authored this study.
Their paper, “Interchange reconnection as the source of the fast solar wind within coronal holes,” was published in Nature on June 7, 2023.
This study was supported by NASA (Contract No. NNN06AA01C). This story does not necessarily reflect the views of this organization.
Original story by Emily C. Nunez: https://cmns.umd.edu/news-events/news/new-study-identifies-mechanism-driving-suns-fast-wind